Ida Noddack: why the first proposal of fission was ignored
How was fission discovered, why was it ignored, and what can we learn from Ida Noddack?
Early in last year’s popular film, Oppenheimer, the young experimental physicist Louis Alvarez is reading the newspaper during a haircut when he abruptly bursts from his chair in a mad dash for the University of California Berkeley Radiation Laboratory in search of the famed Robert J. Oppenheimer. “Oppie! Oppie!” Alvarez shouted, “They’ve done it. They’ve done it. Hahn and Strassmann in Germany. They split the uranium nucleus. They split the atom.” Oppenheimer, for his part, was in disbelief.
Although a slightly dramatized reenactment for the cinema, Alvarez was reading about the discovery of nuclear fission. In January 1939, Otto Hahn and Fritz Strassmann reported surprising evidence for what would be understood as nuclear fission.
In reaction to Oppenheimer, several articles, op-eds, and podcasts rightly noted that this account of the discovery of fission is wanting. Namely, Hahn and Strassmann did not understand the full implications of their experiment. It was their long-time colleague, Lise Meitner—who, being of Jewish ancestry, had fled Berlin—together with her nephew Otto Robert Frisch, that explained the results of Hahn and Strassmann as fission.
The failure to celebrate Meitner’s achievement in Oppenheimer is indeed a sin in the history of science, and hers is one of the most egregious Nobel prize snubs in the award’s history. But in the fervor of critiques, another of the lost women of science, Ida Noddack, fell through the cracks. In a 1934 paper in Angewandte Chemie, Ida Noddack made a radical proposal: when neutrons strike a uranium nucleus, instead of being absorbed to form heavier nuclei, as was the consensus view of the time, the uranium nucleus could instead break apart. The nucleus could fission.
Her prescient conjecture was ignored and ridiculed. And even after Noddack was proven right over four years later, she was not given credit and has since been essentially forgotten. The details of this episode in 1930s nuclear physics demand careful consideration. But before the history lesson, what does it matter?
In the first article of this Seeking Scientific Revolutions blog, I stressed the critical importance of the anomaly. In particular, the anomalous data that is not digested and goes unappreciated but might very well hold the key to a major scientific revolution.
Science today has much to learn from Ida Noddack. Ida was before her time, not only in her genuinely novel and generative scientific ideas—which go well beyond her interpretation of nuclear fission—but also in her demonstrated ability to recognize anomalies and engage them constructively. Ida Noddack has been my scientific inspiration: my scientific heroine if you will. However, this is not the familiar story of an unsung heroine scientist, as in the case of Lise Meitner. As with many brilliant German scientists of the early 20th century, Ida has a dark side.
The details of the nuclear fission origin story
In 1932, James Chadwick discovered the neutron in the lab of Ernest Rutherford, who had predicted its existence twelve years prior. This was a critical milestone. Commonly, nuclear physics experiments used a particle accelerator—like that made by John Cockroft and Ernest Walton in Rutherford’s lab—to bombard different elements with light, positively charged nuclei. But because of the electrostatic repulsion between the incident beam of positively charged nuclei and the positively charged nuclei in the target, the nuclear interactions between the two are rather infrequent. As a neutral particle, the neutron faces no such repulsion.
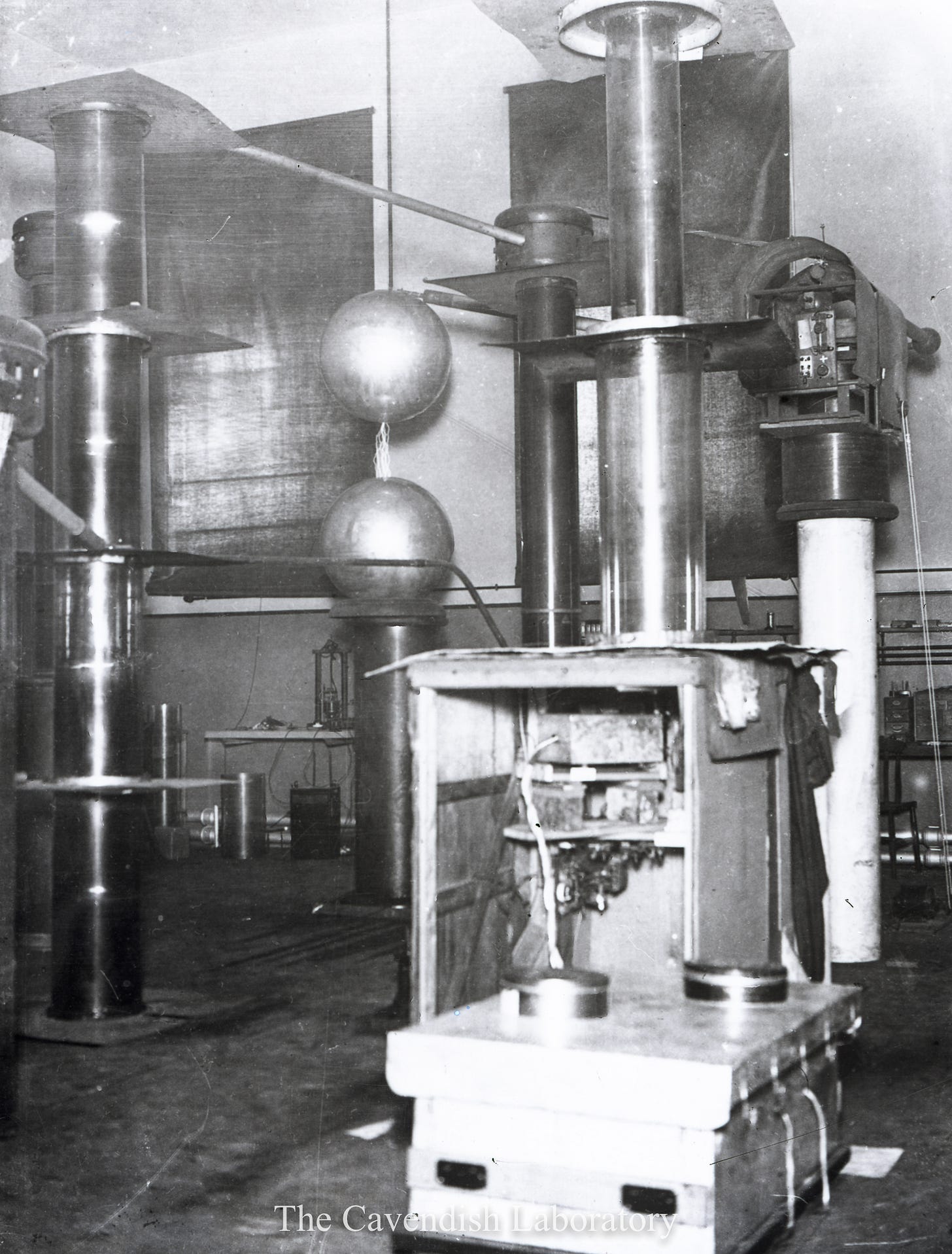
By 1935, scientists had a good estimate of the binding energy (how tightly bound together) of different nuclei, confirming Einstein’s 1905 theory of mass-energy equivalence commonly known by the equation E=mc2. But even still, the scientific consensus was that nuclear energy could not be practically tapped; nuclear physics was merely an academic endeavor.
Physicists had somehow failed to recognize the pattern of nuclear binding energy per nucleon (protons and neutrons) as a function of mass. As nuclei get heavier, the average binding energy per nucleon increases until it peaks at the most stable nuclei (isotopes of nickel and iron) and then declines modestly. Fusing lighter nuclei produces nuclei that are, on average, more tightly bound, requiring less energy to hold the nucleus together. And because of mass-energy equivalence, the resulting nucleus has less mass per nucleon than the two fusing nuclei. That missing mass, being converted to energy, is what we mean when we speak of ‘nuclear energy.’ Similarly, when very heavy nuclei split (fission), the resulting nuclei also have less mass per nucleon, releasing the missing mass as energy. After Lise Meitner and Otto Robert Frisch explained nuclear fission in 1939, the future Manhattan Project contributor Robert Serber remembers reacting, “How could we have been so stupid not to have predicted this?”
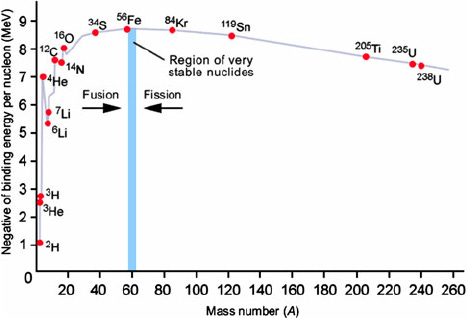
The first splitting of the atom actually came from Rutherford’s lab in 1932 by Walton and Cockcroft splitting a lithium isotope. But as per the nucleon binding energy curve above, it is the fission of heavy nuclei that releases energy.
In early 1934, Irene Joliot-Curie—the granddaughter of Marie Curie—and Fredric Joliot—Irene Curie’s husband and close scientific collaborator—demonstrated the first artificial synthesis of a radioisotope (i.e., artificial radioactivity), where the products of a nuclear transmutation (the process of changing one nucleus into another) remain radioactive after being bombarded by a radiation source. That same year, combining Chadwick’s neutron and Curie’s and Joliot’s artificial radioactivity, Enrico Fermi and his team in Rome bombarded a wide array of elements with neutrons from a radon-beryllium source.
Most notable were Fermi’s experiments on neutron-irradiated uranium, which was then the heaviest known element (uranium has an atomic number of 92), observing artificial radioactivity from its products. Specifically, they reported the discovery of two new elements, element 93 and element 94, which they named ausenium and hesperium, respectively. The accomplished German physicist Lise Meitner was immediately interested in Fermi’s results and, after some convincing, launched a research effort with her long-time collaborator, Otto Hahn, in Berlin.
Here is where Ida Noddack comes into the fission story.
Roughly four months after Fermi’s key papers in Nature on uranium, Noddack—publishing alone as opposed to jointly with her husband, Walter Noddack, and long-time Arbeitsgemeinschaft (a close working partner), which seems to have been their practice when Ida wanted to propose a controversial idea—penned a blistering critique titled “Über das Element 93” or in English “On Element 93.” Noddack noted that Fermi only ruled out elements heavier than lead (element 82) and lighter than uranium—with the exception of polonium (element 84), which was originally ignored by Fermi—to justify his discovery of transuranics.
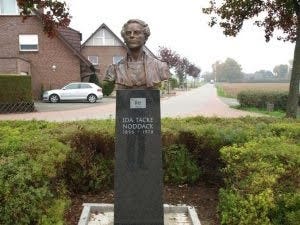
At the time, physicists naively assumed that nuclear transmutations were limited to the ejection of protons, neutrons, and alpha particles (i.e., helium nuclei of two protons and two neutrons) or radioactive beta decay (where the nucleus spits out an electron and an antineutrino or a positron and a neutrino, changing a neutron to a proton or a proton to a neutron, respectively). In other words, a radioactive nucleus could only make small jumps in atomic number. The thinking of Fermi and the rest of the scientific community was that searching such a range of elements was, therefore, sufficient to rule out uranium transmutations that result in a nucleus of lower atomic number, leaving only transuranic elements (i.e., higher atomic number than uranium).
Noddack concluded that the claim to element 93 was incomplete, and while Fermi had stressed that it was “premature to form any definite hypothesis on the chain of disintegrations involved,” the scientific community had readily concluded that the long-sought transuranic elements had been found. In fact, Fermi felt that publicly naming elements 93 and 94 was premature and had kept the names private. The element names were released to the public in a 1934 speech by the physicist and politician Orso Mario Corbino, much to the chagrin of Fermi.
But the most provocative and prescient part of Noddack’s article was her articulation that:
“One could assume equally well that when neutrons are used to produce nuclear disintegrations, some distinctly new nuclear reactions take place which have not been observed previously with proton or alpha-particle bombardment of atomic nuclei. In the past one has found that transmutations of nuclei only take place with the emission of electrons, protons, or helium nuclei, so that the heavy elements change their mass only a small amount to produce near neighboring elements. When heavy nuclei are bombarded by neutrons, it is conceivable that the nucleus breaks up into several large fragments, which would of course be isotopes of known elements but would not be neighbors of the irradiated element.”
The prominent chemists and physicists of the day paid little or no attention to Ida’s critique of Fermi’s analysis and certainly not to her proposed fragmentation of heavy nuclei. In fairness, Ida Noddack did not make the effort to suggest a mechanism by which such a process might occur, given that it would be seen by her colleagues as untenable on the grounds—in hindsight, shaky grounds to be sure—of a lack of energy conservation.
The Noddack’s were skilled element hunters. They had previously reported the discovery of elements 43 and 75. However, the former was widely disputed, and for their continued insistence on its discovery, the pair’s standing fell into disrepute.
In 1935, Fermi’s group demonstrated neutron moderation—the importance of which Lise Meitner proposed in 1934—which is the process of slowing down fast, high-energy neutrons, increasing their chances (i.e., neutron cross-section) of interacting with nuclei. They found that doing so increased specific radioactivity signals, which were identified by their distinct exponential decay.
Parsing the different radioisotopes was difficult work. The best account of this period is Ruth Lewin Sime’s biography of Meitner, Lise Meitner: A Life in Physics, which she synthesized for Physics in Perspective. I draw heavily on her research.
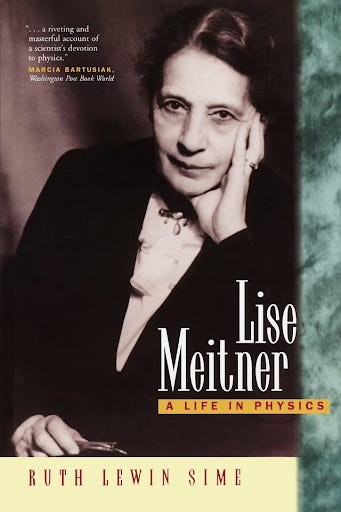
Meitner and Hahn brought on Fritz Strassman, and together, they performed numerous neutron bombardment experiments. Following Fermi’s conclusion in 1934 that there were three distinct uranium disintegration pathways, the Berlin group proposed two neutron capture reactions followed by beta decay, producing transuranic elements (the captured neutron becomes a proton, and the atomic number increases by one). They proposed the third was a neutron capture followed by alpha decay, producing an isotope of thorium. However, there were no observations of alpha particle emission. And per George Gamow’s theory of alpha decay, it seemed implausible that a lone, low-energy neutron impinging on a heavy uranium nucleus could cause the ejection of an alpha particle.
In 1937, Meitner amended the physical interpretation, suggesting that all three pathways resulted from neutron capture by the uranium-238 isotope (uranium is naturally 99.3% uranium-238 abundant) and subsequent beta decay to transuranic species. Expecting the neutron capture to occur most strongly at specific energies (i.e., resonances), as is common with other elements, Meitner moderated the neutron energy in search of a pattern. Confusingly, the first two reaction pathways, which had nominally similar radioactivity and decay times, were more pronounced with slow neutrons, but the reaction pathway was the same for slow and fast neutrons, which is uncharacteristic of a resonance reaction. The third process, which had a longer decay time, only occurred at low energies and not with fast neutrons.
The Berlin team became world renowned for their advanced chemical separation techniques—operating off of the widely-held assumption that the chemical behavior of transuranic elements would be similar to transition metals. As such, the common practice was simply to ignore the filtrate, which proved to be a glaring misstep. They unknowingly filtered out radioactive fission fragments, missing the forest for the trees if you like. Years later, Meitner reflected:
“our misfortune was that we didn't search the filtrate. We couldn't search it because uranium was in it, you know, we couldn't see anything. Our neutron sources were too weak. ... The chemists absolutely didn't want to, I begged them to.”
It is surprising that Meitner was so aware of the trouble in the results and yet when Strassman, as early as 1936, shared that he believed he had observed evidence for barium (element 56)—which would have been then, and later proved to be, a smoking gun for fission—Meitner reportedly disregarded the possibility and the matter was dropped.
Then came a big break. In 1937, Curie teamed up with Paul Savitch, bombarding uranium with slow neutrons. Unlike their German colleagues—and competitors by this point—the French team did not chemically separate the supposed transuranic radioisotopes from the irradiated uranium and instead measured the radioactivity directly. They observed a previously unreported radioisotope that had simply been missed because it was removed in the filtrate of the chemical separation! Interestingly, this new radioisotope could be separated from uranium, which meant it was not an isotope of uranium nor of a transition metal-like transuranic element (otherwise, the Berlin team would have seen it).
In early 1938, the French team managed to chemically separate the radioisotope using a lanthanum salt. And because lanthanum (element 57) shares a column in the periodic table with actinium (element 89)—which means the two elements have similar chemical behavior—they proposed that their beta emitter was actinium. This, however, did not solve Meitner’s theoretical dispute. Indeed, actinium is lighter than thorium, requiring even more nuclear particle ejection.
May 1938 proved to be an important month. Curie and Savitch chemically separated the anomalous radioisotope from actinium, strongly suggesting it was lanthanum. But, of course, lanthanum was considered much too light. In other words, if lanthanum were produced it would tautologically follow that the uranium nucleus breaks apart, as Ida Noddack had suggested. Instead, Curie and Savitch interpreted that their mysterious radioisotope:
“cannot be anything except a transuranic element, possessing very different properties from those of other known transuranics, a hypothesis which raises great difficulties for its interpretation.”
They suggested that the purported transuranic element did not behave like a transition metal but instead as a lanthanide. That same month, Meitner came very close to understanding fission by postulating that perhaps the liquid-drop model of nuclei proposed by Niels Bohr and Fritz Kalckar, adapted from Gamow, was key to making sense of these experiments.
And yet still, despite aligning very well with the voluminous anomalous data, Ida Noddack’s paper was ignored. When Walter Noddack urged Otto Hahn to at least consider Ida’s proposal, Hahn responded by saying that he did not want to embarrass his wife.
Fermi won the 1938 Nobel Prize in physics for “demonstrations of the existence of new radioactive elements produced by neutron irradiation” as well as his discovery of neutron moderation. But, of course, as Ida had argued more than four years before, Fermi had not, in fact, proven the discovery of any new elements.
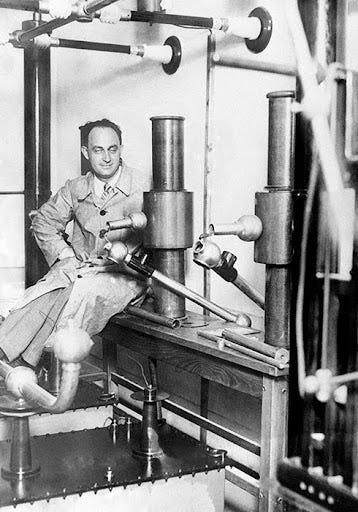
Fermi delivered his Nobel laureate lecture on December 10, 1938, titled Artificial radioactivity produced by neutron bombardment. In it, Fermi proceeded under the incorrect premise that the observed radioactivity from his neutron bombardment experiments resulted from transuranic element production. Nuclei, including uranium, do, in fact, absorb neutrons to some degree, producing heavier nuclei, but Fermi and others actually observed fission products, not transuranics.
Buried in the published version of his Nobel lecture—ostensibly submitted December 12th, according to the date provided on the Nobel Foundation’s website, despite the fact that Hahn had shared his barium results (evidence for fission) only with Meitner and not until December 19th—Fermi adds a footnote:
“The discovery by Hahn and Strassmann of barium among the disintegration products of bombarded uranium, as a consequence of a process in which uranium splits into two approximately equal parts, makes it necessary to reexamine all the problems of the transuranic elements, as many of them might be found to be products of a splitting of uranium.”
Fermi concedes that fragmentation of the uranium nucleus is very much on the table. But Ida Noddack’s name is not mentioned or written once by Fermi!
A week after Fermi’s Nobel ceremony, Hahn and Strassmann sent their manuscript on the discovery of barium to Naturwissenschaften. It was published in January 1939. They had originally believed they had detected radioisotopes of radium (element 88). But their chemical isolation of the radium involved barium compounds! Their methodology had proven their radioisotopes were radium, “if one eliminates barium,” which was assumed at the time to not possibly be produced from neutron bombardment of uranium nuclei. And since barium and radium share the same column of the periodic table as alkaline earth metals, their shared chemical behavior makes distinguishing the two elements a non-trivial task.
It is often rewarding to read original scientific papers of historical consequence for oneself. Hahn’s and Strassmann’s paper is no exception. Theirs is a paper written in the most bizarre fashion.
Hahn and Strassmann proceeded for almost the entire paper, discussing the characteristics of the measured radiation in detail while still referencing the radioisotopes as radium. At nearly the very end of the article, they mention that when they use different barium compounds that concentrate elemental radium with different efficacy, “[radio]activity was distributed evenly among all the barium fractions” and “[radio]activity remained the same for fractions having an equivalent barium content.” Hahn and Strassmann were left with no choice but to conclude that “our ‘radium isotopes’ have the properties of barium. As chemists we should actually state that the new products are not radium, but rather barium itself.” And similarly, the novel lanthanide-like transuranic isotopes reported earlier that year by the Curie-Joliot group “should be lanthanum,” which is far too light to be anything other than a fission fragment of uranium just like barium.
So it is obvious then: the nucleus is fissioning? No.
Hahn and Strassmann continue, “one could not even consider this as a possibility earlier” and in what can be best described as a sort of identity crisis, they finish:
“As chemists we really ought to revise the decay scheme… However as ‘nuclear chemists,’ working very close to the field of physics, we cannot bring ourselves yet to take such a drastic step which goes against all previous experience in nuclear physics. There could perhaps be a series of unusual coincidences which has given us false indications.”
Of course, there is no reference to Ida Noddack’s paper, of which Hahn was fully aware. Per Ida’s account, she had communicated the idea to him directly, and only a few months after the barium publication, Hahn referred to Ida’s paper in a private letter to Meitner.
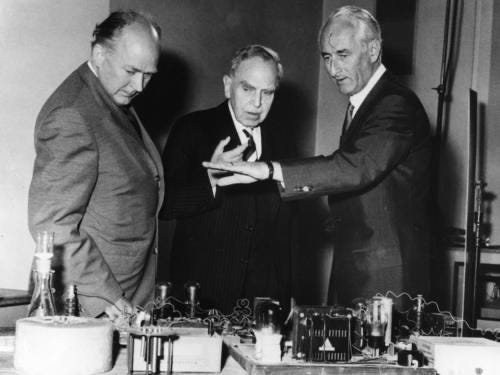
By June of 1938, Meitner had fled to Bohr’s institute in Copenhagen. Meitner and Hahn, though, remained in close contact by letter. Many of these letters have been translated from their original German due to the yeoman’s (F) work of Marissa Moss and Ruth Lewin Sime.
Meitner had been skeptical of the radium results for the same reason she had for thorium: slow neutrons should not be able to cause an emission of alphas as with thorium, let alone two alphas for radium. By Strassmann’s account, she urged that the radium results be “scrutinized very carefully and intensively one more time.’’ A month later, Hahn and Strassmann concluded that the radium was actually barium, and while preparing their manuscript, Hahn promptly reached out to Meitner:
“But we are coming steadily closer to the frightful conclusion: our Ra isotopes do not act like Ra but like Ba… Perhaps you can come up with some sort of fantastic explanation. We know ourselves that it [uranium] can't actually burst apart into Ba.”
Shortly after receiving word of the barium discovery, Meitner and her nephew Otto Frisch tried to make sense of the results. The story goes that while on a walk, the two conceived of how Bohr’s liquid drop model could explain the instability of uranium to fission. Frisch and Meitner both sketched their view of the nucleus splitting in a sort of dumbbell fashion. Below are their original sketches—Meitner’s on the left from a head-on view and Frisch’s on the right from a side perspective. If one of the fragments was barium, by the conservation of charge, the other was krypton, which being a gas, would have escaped Hahn’s experimental apparatus.
Energy conservation still needed to be addressed. In such a fission reaction, the electrostatic repulsion would drive the nuclei apart with 200 MeV of energy. Where might this energy be sourced? Remembering the semi-empirical formula for the curve of binding energy, Meitner did a back-of-the-envelope calculation to determine the ‘missing mass.’ Frisch recalls that when the missing mass was found to be roughly one-fifth the mass of a proton, which, by Einstein’s famous mass-energy equivalence equation of E=mc2, is equal to 200 MeV, “it all fitted!”
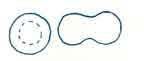
The barium results took the community by storm. In France, Joiliot reportedly immediately understood the implications and began working to confirm the results. Bohr, traveling from Copenhagen, and Fermi, who had fled fascist Italy upon receiving his Nobel Prize weeks earlier, went to share the results at a January conference on theoretical physics in Washington, DC. The paper by Hahn and Strassmann was published during the conference. Bohr intentionally stressed the role of Meitner and Frisch in explaining the results as fission of the uranium nucleus.
Things moved rapidly from this point. Hahn and Strassmann published a follow-up paper verifying their results in February. The next day, the explanation by Meitner and Frisch of nuclear fission was published in Nature and Frisch’s confirmation of the experimental results shortly thereafter. In March 1939, Noddack published a letter in the same journal in which Hahn and Strassmann published their barium results. Noddack chastised Hahn for failing to cite her prediction of nuclear fission and mocked the group’s mistaken transuranics: the proverbial academic ‘I told you so.’
The response—or rather lack thereof—from Hahn and Strassmann is another interesting part of the story that gets told in different ways. The surface-level story is that the editor chose not to publish a very aggressive response from Hahn and Strassmann, opting instead to write that Hahn and Strassmann “have neither the time nor the desire to answer. ... They want their colleagues to be the judges of the correctness of Frau Ida Noddack's demands and the manner of her presentation.” But a closer look at the private communications between Hahn and Meitner in early 1939 suggests another possibility. Despite the widespread adoption of a nuclear fission paradigm, many scientists, including Hahn, still insisted on the discovery of transuranics. But when, around the same time as Ida’s letter, it became clear that the purported transuranic isotopes were actually fission fragments themselves, Hahn could not respond to Ida because he would be forced to admit his error publicly. At this point, Hahn was playing defense, trying to cement his legacy as the discoverer of fission. I suspect he asked the editor to respond. But whatever the backstory, the note successfully dismissed Ida’s claim.
Hahn alone won the 1945 Nobel Prize in chemistry. He failed to give adequate credit to Meitner or to Curie and Joliot, who first uncovered the anomalous fission fragments without fully appreciating their finding. But then again, neither did Hahn, though, as noted by Jeremy Bernstein, he and Strassmann did have the conviction to label their radioisotope as barium and suggest that Curie’s and Savitch’s was lanthanum. A brief note in the published version of Hahn’s Nobel laureate remarks provides a passing recognition—or dismissal, depending on how you read it—of Ida Noddack’s 1934 fission conjecture. And in his 1966 autobiography, Hahn says about Ida’s 1934 paper “Her suggestion was so out of line with the then-accepted ideas about the atomic nucleus that it was never seriously discussed.” But this should be understood as an indictment of the community, not a slight on Noddack. The same year, in a radio interview Hahn admitted, “die Ida hatte doch Recht.” In english: “Ida was right after all.”
Why did Fermi and the physics community miss fission?
How is it that the entire physics community missed nuclear fission for so long? The answer to such a question serves not merely to satiate the committed historian of science but to provide insight as to how today's scientific community might make progress of the same order as the discovery of fission. There are both technical and sociological answers to the question.
Ruth Sime’s book again serves as our guide. You see, in the early 20th century, the search for new elements was a high-status pursuit in both chemistry and physics, and none were more sought after than the transuranics. So the search was on. The neutron bombardment experiments of the 1930s were performed on just about every element the scientific enterprise could get their hands on, but the prize was clear: transmute uranium into new, heavier transuranics.
Also of great interest was the organization of elements, the basis of which was Mendeleev’s periodic table. But at the time, this was by no means fixed; it was a very generative area of research.
In 1934, just before Fermi’s experiments, both Lise Meitner and the younger cavalier Ida Noddack proposed their take on how the elements should be organized. This is important because how one organizes the elements informs expectations of the chemical behavior of those elements. Many expected that the transuranic elements would behave like transition metals.
This expectation from chemistry, paired with the assumption by the physics community that nuclear transmutations should be limited to modest shifts in elemental number, led to a sort of feedback loop. Ruth Sime points out how these false expectations dovetailed with one another and were self-reinforcing: if uranium nuclei captured neutrons producing transuranic elements, they would be situated in the transition metal part of the periodic table and behave like much lighter nuclei. And if then the detected radioisotopes in such experiments were found to be chemically similar to lighter nuclei, they must simply share their chemical behavior but be of a higher-mass transuranic element in the same column of the periodic table.
When Curie and Savitch discovered a lanthanum-like transuranic—which was, of course, actually just lanthanum—the thought was not that of nuclear fragmentation but rather that at least some transuranics actually behave more like rare earth lanthanides than transition metals, which also happens to be true.
Ida: the good, the bad, and the ugly
What is most scientifically attractive about Ida Noddack’s story is that her incisive conjectures went unappreciated. Beyond her interpretation of fission, she prioritized isotopic character (number of neutrons) rather than just elemental character (number of protons/electrons). She was a brilliant chemist; her suggestion was not for a lack of appreciation that chemical activity is driven by the number of valence electrons in an atom. Instead, she essentially predicted that nucleons are organized—analogous to electrons in atomic orbitals—in nuclear shells and that this would conceivably govern the nuclear behavior of isotopes. Moreover she proposed that this should inform our periodic table, now known to nuclear physicists as the Segré chart (Emilio Segré being perhaps Fermi’s most important colleague). The 1963 Nobel Prize in physics was awarded for the nuclear shell model. No, Ida Noddack did not deserve to share this Nobel Prize. I am, however, suggesting that she was incredibly perceptive and demonstrated a track record for being early on big ideas, albeit a little too early.
In 2002, Ernest Hook held a symposium on Gunther Stent’s 1972 article, Prematurity and uniqueness in scientific discovery. The meeting sought a metascientific explanation for periods where science misses something big: a “hang-up” so to speak. The meaning of prematurity—worthy of an essay in its own right—is that scientific ideas that cannot connect with a field’s canon in a few simple logical steps are premature and unlikely to penetrate or affect the direction of said field.
Hook’s main contribution to the proceedings was Interdisciplinary Dissonance and Prematurity: Ida Noddack’s suggestion of nuclear fission. His writing influenced my thinking a great deal. He ultimately finds that Noddack’s fission conjecture was just that: mere conjecture, and that not only did others not pay it any attention, but neither did she. Noddack’s proposal of nuclear fragmentation comes late in her paper and is but one point raised. Hook—as well as other scholars who have taken a close look at this episode—could not find evidence of Noddack working on her proposal after 1934. I think Hook discounts—despite discussing it extensively—Ida Noddack’s effort to gain the attention of the right people. Ida and Walter apparently approached Hahn in 1936, and it seems quite likely that she reached out to Fermi, who, to his credit, apparently made preliminary calculations to gauge the likelihood of Ida’s idea.
Hook inquires, though, why others did not have the presence and openness of mind to take her proposal seriously, pursue experiments, or make calculations. Indeed, I think answering this question holds the most actionable lessons for the scientific community today.
Hook examines several possible explanations. He essentially concludes that the confluence of the Noddack’s political affiliations with the Nazi regime, the couple’s tarnished reputation from their insistence on discovering element 43, Ida Noddack’s tendency to be extremely harsh in her critiques, and coupled with a “relatively minor” case of prematurity explains the lack in uptake of Ida Noddack’s 1934 paper.
I think that is more or less on the right track, with the major caveat that I place a much larger weighting on the prematurity of fission than does Hook. I also question exactly when the Noddack’s reputation for element 43 became so disqualifying. Per an American Institute of Physics interview with Emilio Segré in 1967, he had only come to feel that the Noddack’s claim to element 43 was “dishonest” in 1936 or 1937, two or three years after Ida’s 1934 paper. He remembers, “And we [Fermi’s team in Rome] saw the reprint [Ida Noddack’s paper]. I remember this reprint as if it were now.” Segré further recounts speaking with Hahn in the 1960s, confirming that Hahn had indeed seen Ida’s paper, and asking why he had not paid it any attention, to which Hahn apparently replied, “I don’t know.” Segré, too lamented, “And I don't know why we didn't pay any attention to it in Rome. I really don't.”
Later in the interview, unprompted, Segré circled back to Ida Noddack:
“But if somebody had thought that there was fission, he wouldn't have blocked them off [added radiation shielding blocking out the fission fragment radiation]. Now, why was Ida Noddack so totally rejected by everybody?... I'll tell you. It's a question for which there is no answer because I asked Fermi and he was very cagey. At least I couldn't elicit any clear answer from him. ‘Why didn't you think of fission? When you read Ida Noddack—what were you, asleep?’ As far as I am concerned, personally, I would say I was too stupid, which is not an answer. It begs the question.”
I think Segré’s view is quite valuable. He was no fan of the Noddacks; he was a Jewish refugee and thought they were Nazis. Yet in another interview, 16 years later, Segré was even more emphatic:
“Because I asked Hahn, I asked Joliot [Curie] and so on. I know for sure in Europe—I know that in at least three places, it was noticed and read. I’m practically sure, although I didn’t ask specifically, whether [Ernest] Rutherford or [Sir James] Chadwick or somebody at Cambridge [University] read it, but I’m practically sure that somebody read it. Fermi, he wrote that the reaction was—that no, it can’t split. But I don’t know why it was said so… I don’t believe that at that time Aston’s curve for the mass defect [curve of binding energy per nucleon] would not have given a minimum with the two fragments [fission]. I’m practically sure of it. I haven’t rechecked on the Aston publication at the time.”
The Harvard professor of the history of science Naomi Oreskes took umbrage at Hook’s conclusions, arguing in Science for gender bias as the key factor. Her argument is without merit, as is her incorrect critique of the prematurity hypothesis more broadly.
The reasoning is pretty straightforward. For one, it is quite unlikely that Meitner or Curie—both widely respected female scientists, Curie having won the 1935 Nobel prize in chemistry for artificial radioactivity—would have dismissed Ida’s ideas because of her gender. Hook and I agree that it is also hard to believe that many of the relevant male players in this story (e.g., Fermi, Hahn, Strassman, Joliot, Bohr, etc.) would have been so ignorant, either. After all, quotes abound of Hahn and Strassmann clearly differing to Meitner’s physics prowess. Moreover, Ida and Walter Noddack were together nominated numerous times for the Nobel Prize in chemistry for their discovery of element 75. Hook’s response, also in Science, additionally points out that Oreskes quotes the chapter in a misleading fashion, suggesting that Hook—and presumably anyone who shares his view after careful consideration of the historical evidence—is ignorant of misogyny in 20th-century science. Much to the contrary, my expectation going into my research—and Hook’s expectation as well, as he explicitly mentions in his chapter—was that Noddack’s gender was the likely explanation for her dismissal. Indeed, misogyny was the reason why Ida Noddack did not have a formal academic post. But of course, one actually has to do the work and dispassionately assess the evidence, which Hook’s chapter does very thoroughly.
Oreskes approached the Ida Noddack story with a preordained heuristic in search of a Meitner-like heroine. But that is not this story. Oreskes does not like the idea of prematurity in science and alleges that those who suggest it plays an important role in cases such as Ida’s are “unduly reductionist,” which is ironic given that her critique only lists gender bias as a “factor.” After Hook’s counter, Oreskes performs an about-face, asserting that she merely suggested that gender bias happens to be one of many factors that played a role in Ida’s story.
A scientific heroine of mine for her bold and heterodox ideas, Ida Noddack really did appreciate the importance of anomalies. So it stung extra hard to learn of her, in the very best case, fraught relationship with national socialism and the Nazi academic complex. She and Walter were officially exonerated of Nazi affiliation during denazification in the post-war period. But they struggled to gain scientific jobs because it was widely felt in the scientific community that they had embraced Nazi rule.
Indeed, Walter Noddack replaced a Jewish chemistry professor in 1933 despite protest from a fellow academic. Moreover, Emilio Segré recounted that Walter had visited him in Italy in 1937 to discuss element 43, wearing “an irregular military uniform complete with swastikas.” For Ida’s part, her only formal academic position came during a two-year stint from 1942 to 1944 at the Nazi regime’s prized Strasbourg University, infamous for its Aryan pseudoscience.
Jeremy Bernstein provides the categories of Nazi, non-Nazi, and anti-Nazi. He concludes that the Noddacks were at best non-Nazis, as Hahn was—that is, not true believers in Nazi fascism and not members of the Nazi party, but nevertheless willfully ignorant of Nazi atrocities and complicit in their silence. In contrast, Strassmann was an anti-Nazi; he hid Jews during the war. The disdain for the Noddacks after the discovery of fission was almost certainly in large part for their reprehensible embrace of the Nazis.
What kind of novel ideas should we care about?
The collective effort to discover fission spans multitudes. There were several key efforts and contributions. The point is not to pontificate about who should have won the Nobel Prize. However, since I cannot help it, my sense is that the Nobel Prize in physics should have been shared by Meitner, Fermi, and Frisch and in chemistry by Hahn, Strassman, and Curie. All six of these individuals made key scientific insights and experimental findings. No, Ida Noddack did not deserve a Nobel Prize for her conjecture; it was not robust, nor did it actually explain the proposed process.
So what is the point? Why do I harp on Ida’s contribution if it ultimately proved not to be influential on the community?
Because there is an important lesson for today’s scientific community, even more so than for the history of fission. Ida’s idea was novel and proved to be spot on. It was heretical, incomplete, not even rigorous, and just downright offensive. But a scientific community that can, in the moment, recognize an Ida, be attuned to the insight presented even if half-baked (or less) is one which can make progress much more rapidly and is less likely to miss the important anomalies as Fermi, Hahn, Meitner, Strassman, Curie, Joliot, Bohr, and so many others all failed to see for so long.
For our sake and that of so many in Europe, we should be grateful that the scientific community of the 1930s failed to heed Ida’s insight. For if fission had been discovered sooner, perhaps the Nazis would have beaten the Allies to the bomb. Perhaps it is a word of caution to scientists working on genuinely novel work to be mindful of its potential consequences. But ultimately, forward is the only way; and the greatest scientific discoveries progress one anomaly at a time.